Medical Imaging Types and Modalities
Published: 13 April 2021
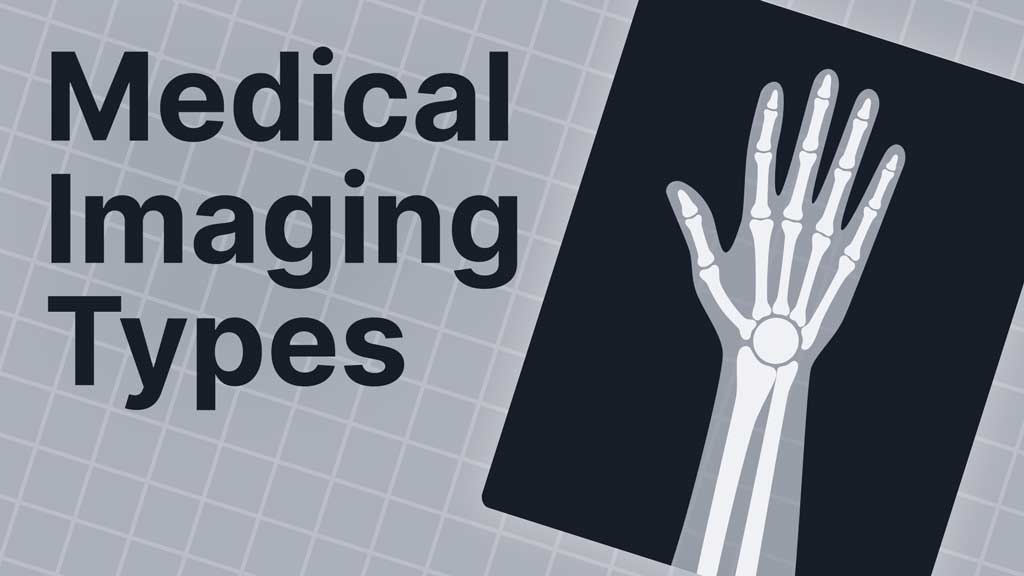
Published: 13 April 2021
In an ideal world, we’d be able to diagnose and treat patients without any harmful side effects. Medical imaging remains one of the best ways to achieve this, as it allows us to see what’s going on inside the body without the need for surgery or other invasive procedures. Indeed, it’s something we’re perhaps guilty of taking for granted at times.
Medical imaging can be used for both diagnosis and therapeutic purposes, making it one of the most powerful resources available to effectively care for our patients.
In terms of diagnosis, common imaging types include:
Each type works slightly differently to create images of what’s going on inside the body. Let’s look at them a little closer.
Ultrasound is a typically non-invasive and safe form of medical imaging that has a wide range of applications (NPS MedicineWise 2019).
Ultrasound uses sound waves rather than ionising radiation. High-frequency sound waves are transmitted from a probe to the body via conducting gel. Those waves then bounce back when they hit the different structures within the body, creating a real-time image (FDA 2020a).
Another type of ultrasound commonly used is the ‘Doppler’, which allows the blood flow through the arteries and veins to be visualised (FDA 2020a).
Due to the minimal risk associated with Ultrasound imaging, it’s the first choice for viewing the fetus during pregnancy (FDA 2020a). However, as its applications are so wide, it tends to be the first port of call for many patients. Ultrasound imaging can be used to diagnose conditions in other areas of the body, including:
(NPS MedicineWise 2019)
X-ray imaging is the oldest but one of the most frequently used imaging types (RadiologyInfo.org 2018).
Discovered back in 1895, X-rays are a form of electromagnetic radiation. X-rays work on a wavelength and frequency that we’re unable to see with the naked, human eye. However, they can penetrate through the skin to create a picture of what’s going on beneath (History.com 2020).
While typically used for diagnosing issues affecting the skeletal system, X-rays can also be used to detect other issues such as breast cancer (through mammography) and digestive issues (through barium swallows and enemas) (FDA 2020b; Better Health Channel 2019).
X-rays are widely used as they are quick and relatively easy for the patient to endure. However, there are risks associated with the use of radiation for X-ray imaging.
Every time a patient undergoes an X-ray, they receive a dose of ionising radiation. This may go on to cause issues such as:
(FDA 2020b)
Most of these risks are mitigated by only using X-rays when strictly necessary, and correctly shielding the patient's body.
Computer tomography (CT) (also known as computed axial tomography (CAT)) uses X-rays to produce cross-sectional images of the body (NPS MedicineWise 2019). The CT scanner has a large circular opening so that the patient can lie on a motorised table. An X-ray source and a detector then rotate around the patient, producing a narrow ‘fan-shaped’ beam of X-rays. These X-rays pass through sections of the patient’s body in order to create 'snapshots', which are then collated into one or multiple images of the internal organs and issues (FDA 2019).
CT scans provide greater clarity than conventional X-rays, producing more detailed images of the internal organs, bones, soft tissue and blood vessels within the body (FDA 2019).
The benefits of using CT scans far exceed the risks, which (like with X-rays) include an increased risk of cancer, harm to an unborn child or a reaction to the contrast agent or dye used. In many cases, the use of a CT scan prevents the need for exploratory surgery (FDA 2019).
It is crucial that when scanning children, the radiation dose is lowered (ACSQHC n.d.). You’ll find a paediatric CT scanner in many hospitals for that reason.
Magnetic resonance imaging (MRI) creates diagnostic images without using harmful radiation (NPS MedicineWise 2019).
MRI uses a strong magnetic field and radio waves to generate detailed images of organs, soft tissues, bones, ligaments and cartilage (NPS MedicineWise 2019). It is commonly used to examine internal body structures in order to assess:
(FDA 2017; MITA 2021)
As we know, the human body is made mostly of water, and each water molecule contains a hydrogen nucleus (proton). An MRI scanner uses a strong magnetic field to force the protons to align to that field. A radio frequency is then applied, which stimulates the protons and spins them out of equilibrium. When the frequency is turned off, the protons return to their original alignment, releasing energy that is detected by the MRI sensors (NIBIB 2013).
Protons in different body tissues return to their normal alignment at different rates, allowing the MRI to distinguish between various types of tissue and identify any abnormalities. The way in which the molecules ‘flip’ and return to their normal spin alignment is recorded and processed into an image (NIBIB 2013).
MRI doesn’t use harmful ionising radiation. However, there are other disadvantages associated with its use.
As strong magnets are used, metal implants such as pacemakers and implantable cardioverter-defibrillators (ICDs) in the patient's body can be hazardous as they may be moved or heated up within the magnetic field (Cleveland Clinic 2017). There have been several reported cases of patients with pacemakers dying through the use of MRI (Nordbeck, Ertl & Ritter 2015). Other disadvantages associated with MRI include:
(FDA 2017; NPS MedicineWise 2019)
A positron emission tomography (PET) scan involves injecting, swallowing or inhaling a radioactive agent known as tracer. The patient then lies in a PET scanner, a large doughnut-like structure that detects gamma rays from the tracer and converts them into images. Bright spots on the image indicate higher chemical activity, potentially caused by a disease (Healthdirect 2019).
PET scans are used to diagnose conditions such as tumours, heart disease and brain disorders. They can be used to assess the entire body or one specific area (Healthdirect 2019).
While PET scans are generally painless for the patient, they involve exposure to ionising radiation. Other disadvantages include the risk of adverse reactions to the radioactive material and potential feelings of claustrophobia for the patient (NPS MedicineWise 2019).
One thing we do have to be aware of as medical professionals in a time of escalating medical costs and increasing demand is that we’re using the best resources available to meet the needs of our patients. This means making a careful decision about the right medical imaging for each patient and their potential diagnosis.